The Growing Role of Nanotechnology in Neurological Medicine
Nanotechnology is revolutionizing neurological medicine by enabling precise drug delivery and treatments. Techniques like PLGA and Cadmium Selenide nanoparticles bypass the blood-brain barrier, targeting conditions such as Alzheimer’s disease. With methods like lithography and vacuum deposition, the
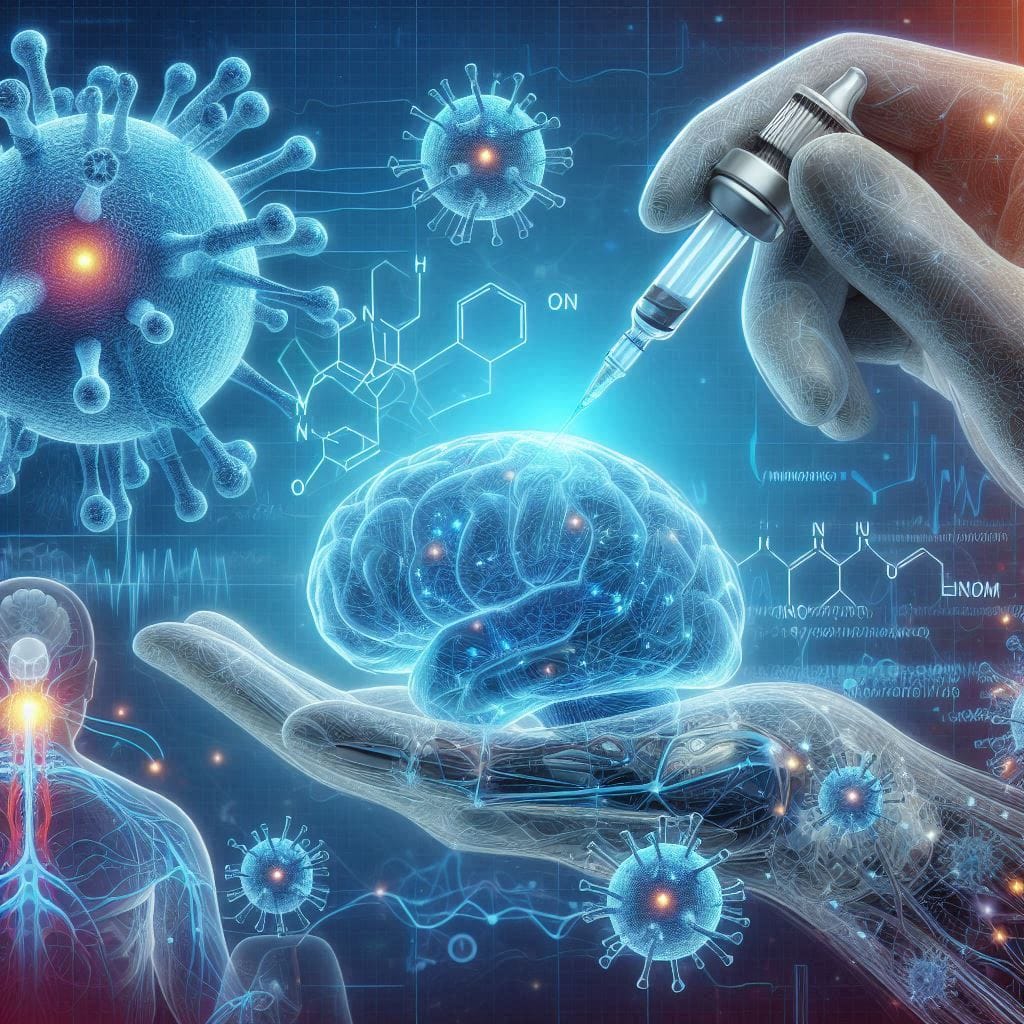
Legacy articles aren't reviewed and may be incorrectly formatted.
The Growing Role of Nanotechnology in Neurological Medicine
Colquhoun, Archibald (EJNR)
Nanotechnologies are particles, normally at an atomic and molecular scale, that are manipulated to create new structures that we can use specifically at sizes smaller than 100nm. These are often versatile and useful particles that can be catered to fulfill exact needs, allowing our creation of materials that are very efficient and effective at a given role. A key property of these technologies is their innately high surface area to volume ratio, resulting in a high potential to create both reactive molecules and efficient agents. This property has led to our abilities to create smaller and more effective electronics and energy storage devices as well as more, useful materials. When applied to humans, nanotechnology has enhanced our ability to catalyse metabolic prossesses, image diseases, clot blood and deliver specifically acting drugs to their targets. Despite potential problems it could cause, such as bioaccumulation and potential ethical issues raised, by having invisible particles that could potentially be deadly or be used to intrude on peoples privacy, nanotechnology has potential to revolutionise medicine and enable doctors to cure disease in more areas that were previously less accessible, such as in the brain. Nanotechnology has allowed us to efficiently deliver drugs past the blood brain barrier, which stops many drugs from acting in the brain, which permits us to aid people with neurological disease more often and more efficientlu than before it was developed.
In the 1950’s, people started using techniques such as lithography to engrave molecules, utilising methods such as photolithography (engraving using light) and electron beams to achieve a desired shape. This initial nanotechnique lead to the pioneering of new techniques to create useful particles, such as etching. This technique uses agents such as nitric acid to destroy parts of particle (this method is often used after lithography has been used to remove a protective coating on a particle, so the acid destroys unprotected parts of a particle, achieving a more detailed, desired shape). Another kind of technique which is commonly used to synthesize nanotechnologies is deposition. One such technique is vacuum deposition, which involves coating a particle in a gaseous molecule in a vacuum. The advantage of vacuum deposition, pioneered in 1970, is that it avoids impurities in the coating of a substance. As more of these techniques are devloped, it allows scientists to create new techniques of targetting disease and create particles with more specific and effective roles.
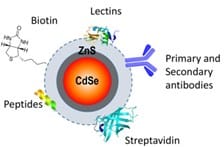
Cadmium Selenide is a molecule created by a technique called self assembly. It involves using electric fields to induce a charge across the atoms in a mixture of Cadmium and Selenium, known as dipoles. This results in the atoms attracting and reacting to form Cadmium Selenide (CdSe) molecules. The CdSe is coated in a layer of Zinc Sulphide to increase biocompatability by containing the toxic ion of Cadmium. This molecule is used both in solar panels, to transfer photons from solar radiation into electrical energy, as well as to biologically label cells by bonding to various components of the cell membrane including cholesterol. An important property of nanotechnologies in the body is their accumulation in damaged/leaky tissues, known as the enhanced permeabiliy and retention effect. CdSe utilises the EPR effect and can be used to highlight damaged areas of the body, including cancerous tumors, making this nanotechnology particularly useful in detection and diagnosis of disease.
This is done by coating the surface in primary antibodies, which are designed to attach to both the Zinc Sulphide coating of the CdSe and a secondary antibody. This secondary antibody binds to the primary antibody as well as an antigen on target cells. This has allowed Cadmium Selenide to be used in the marking of liver cells. Other molecules such as biotin (vitamin B7) and peptides can be attached to the surface to help target specific areas of the body, such as cancer cells. This demonstrates the promise of new nanotechnologies being developed that can be used in medicine.
Cadmium Selenide is an effective nanoparticle for the detection of disease because of the ease with which it can be detected and traced through the body. It also avoids any radioactive isotopes, unlike the more commonly used technique of PET scans, which use positron-emitting isotopes that can cause cancer. The main issue is that, despite the Zinc Sulfide coat, it is still difficult to say how biocompatible it is and therefore how safe it is to use in humans.
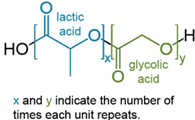
Polylactic-co-glycolic acid (PLGA) is a molecule which combines two compounds which were previously used for similar purposes: polylactic acid and polyglycolic acid. PLGA is used to deliver drugs to specific parts of the body by coating the surface of the drug in PLGA molecules and has shown promise in other roles such as tissue scaffolding. PLGA can be administered by various methods, mainly intravenously, however it can be administered by swallowing tablets, through creams as well as into the spinal cord and the cerebrospinal fluid. To prepare a drug, the drugs surface is coated in PLGA, which breaks off the drug to deliver it within the body. This coating is formed by the condensation reaction of lots of PLGA molecules, losing H2O (the atoms in black ink in the diagram) to form an ester bond between lactic acid atoms and glycolic acid molecules. PLGA is fully biodegradable and can be fully broken down by the body into lactic and glycolic acids, which means it can be put into the body in reasonable quantities with no risk of poisoning. The efficiency of this depends on the ratio of Lactic acid to glycolic acid in the PLGA coating of a molecule.
The lower the ratio i.e. the more glycolic acid, the easier it is for the body to metabolise the PLGA. This is due to the molecule being (overall) more hydrophilic because there are more polar parts of the molecule. This occurs because the more electronegative oxygen makes the Carbon atom it is bonded to slightly positively charged. With no methyl group attached, this charge is spread out less in glycolic acid, resulting in a stronger charge (while still being a fraction of a positive charge on the carbon atom), resulting in a more polar molecule. Further, there are no non-polar, hydrophobic methyl groups on Glycolic Acid. This more polar structure of glycolic acid allows enzymes such as Proteinase k and Trypsin to act on it more efficiently.
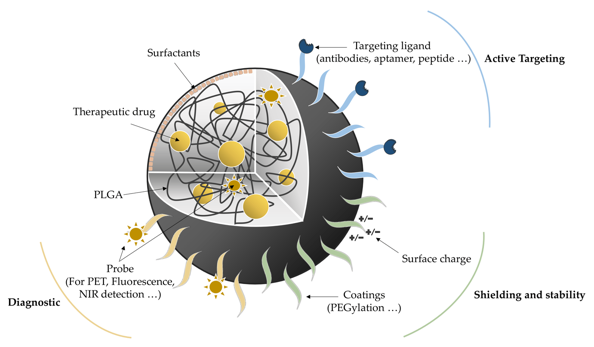
To encapsulate a drug in PLGA, there are a few methods. The simplest begins by dissolving the PLGA and a drug in an organic solvent (e.g. dichloromethane) before emulsifying the mixture by adding an aqueous phase (a solution). This combines the drug and PLGA. The final step then requires them to be precipitated out of solution. This process of nano-precipitation is achieved by either doing a single or double emulsion, depending on the solubility of the drug and the PLGA. If they are more soluble (have a lower ratio of lactic to glycolic acid i.e. more, more soluble glycolic acid in the coating), then an oil-in-water (O/W) emulsion is used by mixing the aqueous phase with an oil such as chloroform. For a double emulsion, a water-in-oil-in-water is required (W/O/W) which follows the same steps as for an O/W emulsion, before dissolving in a final aqueous phase, e.g. polyvinyl alcohol. Whilst being emulsified, the mixture is being heated and stirred until eventually all the solvents are evaporated.
The PLGA-drug nanoparticles can also be synthesised using electric fields by mixing the drug and PLGA in a solvent and pumping the mixture through a capillary tube. An electric field is created around the tube, the energy of which overcomes the hydrogen bonds between solvent molecules, reducing the ability of the solvent to dissolve the solute and precipitates the nanoparticles. The advantage of this method is that it is much more effective at encapsulating the drug than emulsion solvent methods with a 99% average yield of properly encapsulated drugs which compares to an average of 30% in the other method, however it is much less efficient for mass production of PLGA-drug nanoparticles.
This PLGA coating can have surface molecules attached to it to achieve a desired outcome. Some examples of these molecules can be targeting molecules like antibodies or peptide chains so that the nanoparticle will act in a specific area of the body. Isotopes of atoms can be added to some of these molecules so PET scans can highlight areas where the nanoparticle accumulates, e.g., a specific cell type. Polyethylene glycol can be added makes it harder for your body to recognise the antigens on the drug as foreign, reducing the chance that nanoparticles will be intercepted by the immune system.
Desired surface molecules can be added by re-emulsifying the PLGA encapsulated drug after it has been synthesised after adding it to an aqueous phase. This ensures that the surface molecules end up on the surface of the PLGA, which might not happen if the molecules were added at the same time as the PLGA.
PLGA is an effective nanotechnology in medicine because it is versatile, thanks to the range of surface molecules it can have. It is also safe, due to its ability to be metabolised by the body safely, and it is a stable method that maintains the integrity of the drug it carries.
The downsides of PLGA, however, are that it has a complex manufacture method, making it hard to mass produce PLGA nanoparticles. This results in expensive and low quantity products, that may not be effective for patients that need drugs that have to be administered often. The rate of release is still quick, despite the action of the PLGA, so it does not significantly decrease the frequency with which a drug needs to be taken and most of the nanoparticles are broken down quickly, so it is not more effective than simply administering a drug without a coating in most circumstances.
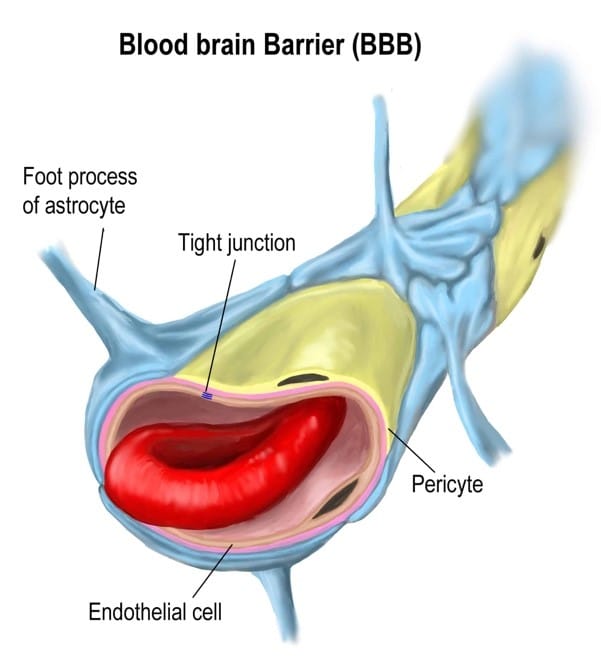
Polylactic-co-glycolic acid (PLGA) is a molecule which combines two compounds which were previously used for similar purposes: polylactic acid and polyglycolic acid. PLGA is used to deliver drugs to specific parts of the body by coating the surface of the drug in PLGA molecules and has shown promise in other roles such as tissue scaffolding. PLGA can be administered by various methods, mainly intravenously, however it can be administered by swallowing tablets, through creams as well as into the spinal cord and the cerebrospinal fluid. To prepare a drug, the drugs surface is coated in PLGA, which breaks off the drug to deliver it within the body. This coating is formed by the condensation reaction of lots of PLGA molecules, losing H2O (the atoms in black ink in the diagram) to form an ester bond between lactic acid atoms and glycolic acid molecules. PLGA is fully biodegradable and can be fully broken down by the body into lactic and glycolic acids, which means it can be put into the body in reasonable quantities with no risk of poisoning. The efficiency of this depends on the ratio of Lactic acid to glycolic acid in the PLGA coating of a molecule.
The lower the ratio i.e. the more glycolic acid, the easier it is for the body to metabolise the PLGA. This is due to the molecule being (overall) more hydrophilic because there are more polar parts of the molecule. This occurs because the more electronegative oxygen makes the Carbon atom it is bonded to slightly positively charged. With no methyl group attached, this charge is spread out less in glycolic acid, resulting in a stronger charge (while still being a fraction of a positive charge on the carbon atom), resulting in a more polar molecule. Further, there are no non-polar, hydrophobic methyl groups on Glycolic Acid. This more polar structure of glycolic acid allows enzymes such as Proteinase k and Trypsin to act on it more efficiently.
PLGA does, however manage to overcome the blood-brain barrier (BBB), resulting in a new ability for drugs to reach the brain and the ability to heal neurological disease. The purpose is to stop toxins reaching the brain and to allow for a more sterile and nutrient specific medium for neural activity to occur in.
The specialised, key component of the BBB is the basement membrane which is inbetween the capillaries and the brain. It filters what passes from the blood into the brain. This is what stops most drugs entering the cereberospinal fluid (CSF). There are a few different routes that nutrients from the blood and waste products from the brain take to cross the barrier.
The simplest is passive diffusion, which just requires molecules to diffuse through the membrane or around endothelial cells. This is the route oxygen and carbon dioxide take, as well as many anasthetics, for example nitrous oxide, which are used in medicine. Most drugs are too large to ustilise this route. Larger molecules, such as glucose, have specific proteins that pump molecules past the barrier, such as GLUT1 proteins. This does not work for drugs because the body does not have specifically shaped pumps for the drugs, so they cannot be pumped through by the body. The other route is by entering the endothelial cells via endocytosis, the process of cells taking up molecules from extracellular spaces such as in the blood. The cells normally need to be triggered to start this route, meaning drugs rarely take this route. This is the route that PLGA encapsulated drugs mostly take. On the surface of PLGA, molecules can be added that will bind to receptors on cells in the blood brain barrier, resulting in endocytosis. The cell then does transcytosis – moves the nanoparticle across the cell – and upon doing exocytosis offloads the drug into the CSF.
The rate that PLGA nanoparticles cross the BBB can be sped up by reducing their size. This is due to an increased rate of cellular uptake, because it is easier for the cell to do endocytosis with smaller particles, and larger surface area to volume ratio, resulting in more surface molecules to trigger endocytosis. Increasing the number of surface molecules on the nanoparticle that trigger the endocytosis by increasing the number of them added while synthesizing the nanoparticle is an effective method of increasing the rate of uptake.
Finally, the use of PLGA nanoparticles in conjunction with other therapies can increase the rate of uptake. Focused ultrasounds (FUS) are a non-invasive technique which involves injecting microbubbles of gas into the bloodstream, which are then exposed to ultrasound waves. This excites the gas, creating gaps between endothelial cells that nanoparticles can diffuse through and enhances the permiablitity of the basement membrane. Normally, gaseous bubbles in the blood stream are quite dangerous because they can cause embolysm, a potentially deadly condition, however if the bubbles are managed both in number, size and composition (non-toxic gases) the risks of embolysm can be minimized. Some of the problems with FUS are that they can cause tissue damage, have limited depth of efficacy and are quite complicated to do, both due to the specialist equipment required and to achieve the accuracy required. In severe circumstances, however, these risks can be worth it to help deliver drugs to the brain.
An example of such a severe brain condition is the neurodegenerative disease alzheimers. This occurs when tau proteins, which are important in supporting the structure of neurons, mutate and cause lots of phosphate groups to bind to them, known as hyperphosphorylation. This results in less stable neurons. In conjunction to this, amyloid protein plaques form as more proteins mutate and disrupt neural function. This triggers an immune response in the brain, causing inflammation, which can damage the brain due to excessive swelling.
PLGA can be used to deliver drugs that help by, for example, clearing amyloid plaques, e.g. rifampicin, or help mitigate the effects of the disease. This can help stop brain function deteriorating and maintain cholinergic activity – the kind of neural activity that is active in learning as opposed to activity such as recalling. Drugs that reduce inflammation, e.g. curcumin, reduce neuron damage, so if PLGA is used to deliver such drugs we can help people suffering from the disease. The PLGA targetting antibodies can also be used to target the drugs to the hippocampus, the part of the brain relevant to memory, enabling us to help retain diseased people’s memory.
Another therapy that PLGA capsules have enabled is the insertion of siRNA into cells which produce mutated tau proteins. Small interfering RNA can stop the expression of genes that code for the proteins, and by using PLGA to drop them off, they can be inserted into neurons and can cure them of that part of Alzheimers disease. This is a treatment that is being tested as a potential cancer treatment, which uses other nanotechnologies to encapsulate the drug similarly to PLGA, and relies on similar mechanisms that PLGA has such as the same targetting methods.
The potential of PLGA and other nanotechnologies to solve problems that have been so far unsolvable cannot be ignored. As nanotechnology develops with new methods of nanofabrication, we will be able to revolutionize medicine and improve both the quality of life for patients and our ability to help them. Our ability to both to diagnose more accurately and efficiently as well cure diseases that currently are impossible to help will develop as more and more specific treatments are developed.
Bibliography
- https://www.nanowerk.com/The-Different-Types-of-Lithography-in-Nanotechnology.php#:~:text=In%20its%20modern%20form%2C%20lithography,sensitive%20material%20called%20a%20photoresist
- https://www.xometry.com/resources/blog/dry-etching-vs-wet-etching/#:~:text=Generally%20speaking%2C%20in%20wet%20etching,related%20to%20their%20corrosive%20properties
- https://www.sciencedirect.com/topics/chemistry/cadmium-selenide-nanoparticle#:~:text=CdSe%20nanoparticles%20have%20been%20extensively,107%20and%20solar%20energy%20conversion.&text=Furthermore%2C%20CdSe%20has%20a%20bandgap,tuned%20across%20the%20visible%20spectrum
- https://www.sciencedirect.com/science/article/pii/S0264127522005123
- https://akinainc.com/polyscitech/PLGA/PLGA.php
- https://www.sciencedirect.com/science/article/pii/S1549963420301532
- https://link.springer.com/article/10.1007/s40204-020-00139-y
- https://link.springer.com/article/10.1186/s11671-016-1509-3
- https://altoida.com/blog/the-function-of-blood-brain-barrier-and-neurological-diseases-how-they-work-together/
- https://www.mdpi.com/1999-4923/14/1/214
- https://www.ncbi.nlm.nih.gov/pmc/articles/PMC4292164/
- https://onlinelibrary.wiley.com/doi/full/10.1002/advs.202003937
- https://www.degruyter.com/document/doi/10.1515/ntrev-2012-0084/html?lang=en
- https://www.ncbi.nlm.nih.gov/pmc/articles/PMC8067506/
- https://www.mdpi.com/2076-3417/11/9/4305
- https://onlinelibrary.wiley.com/doi/10.1155/2015/692672
- https://www.ncbi.nlm.nih.gov/pmc/articles/PMC5655257/
- https://www.frontiersin.org/journals/pharmacology/articles/10.3389/