Sonodynamic Therapy: A Cutting-Edge Approach in Cancer Treatment
The struggle against cancer is one of humanity’s greatest challenges, but hope is on the horizon. This article dives deep into a rapidly-evolving cancer treatment method which utilises ultrasound coupled with targeted drugs to selectively kill cancer cells without causing systemic toxicity.
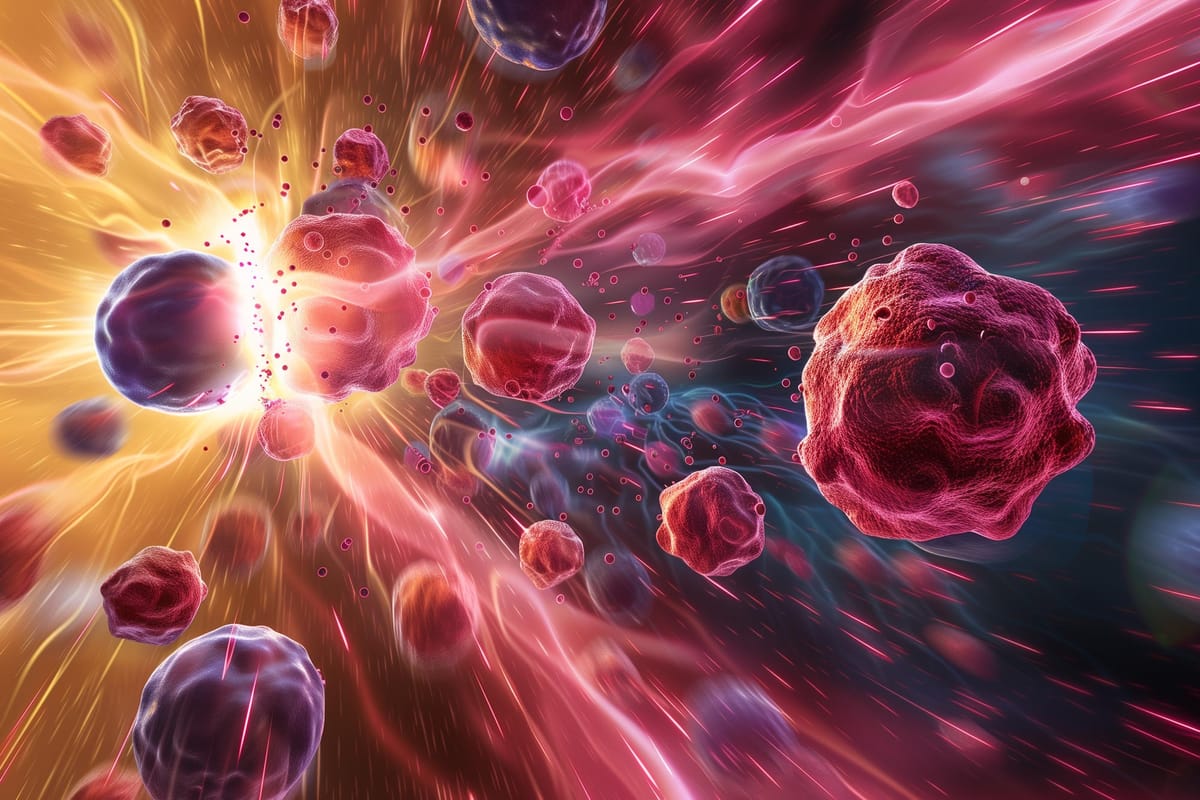
Introduction
As we know, cancer has become the leading cause of mortality globally, and anticancer therapy has attracted more and more researchers’ attention. Current cancer treatment methods are reliable and clinically applied, but every kind of treatment has its own limitations which make rigorous challenges for cancer therapy. Sonodynamic therapy is a non-invasive method of anti-cancer treatment that utilises ultrasound. Ultrasound is a type of mechanical sound wave with frequencies of >20 kHz, with a high penetration depth of >10cm in soft tissues. Inspired by photodynamic therapy, it works by blasting a high-intensity ultrasound beam to destroy and denature a targeted tumour. Existing cancer treatment strategies cause systemic toxicity or cannot penetrate deep tissue to effectively stop tumour metastasis, making sonodynamic therapy an efficient alternative with fewer side effects. Among emerging therapies, it stands out as a novel, minimally invasive cancer treatment that combines ultrasound waves with chemical agents to selectively target and destroy tumour cells. First developed in the 1980s, It has evolved into a powerful tool against hard-to-treat cancers. This article presents the mechanisms involved in sonodynamic therapy and its applications.
Mechanisms Involved in Sonodynamic Therapy
Solid tumours, compared to normal tissue, exhibit enhanced permeability and retention (EPR), allowing nanoparticles to selectively accumulate at the tumorous site (Figure 1). Sonodynamic Therapy (SDT) alone often has limited efficacy against tumours, but it becomes highly effective when coupled with sonosensitisers—chemical drugs that induce tumour necrosis (cell death). Sonosensitisers, pre-administered with microbubbles (microscopic gas bubbles 1-10 μm in diameter), can remain in cancer cells for extended periods due to the EPR effect, selectively accumulating in tumours while gradually diffusing out of healthy tissue within 24-72 hours post-administration.
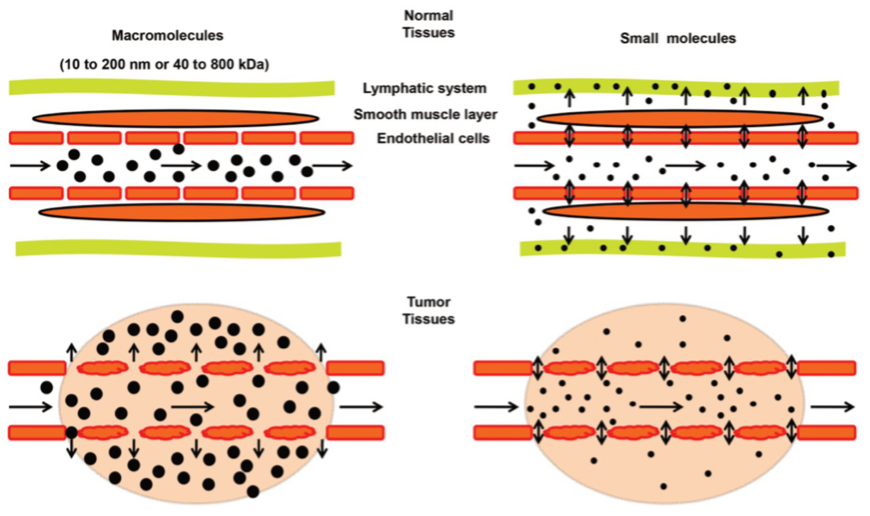
The mechanism of SDT involves the use of low-intensity ultrasound to create a cytotoxic effect through focused mechanical waves. Under ultrasound exposure, microbubbles undergo cyclic expansion and contraction in a process called cavitation, inducing rapid temperature and pressure changes in cells, providing energy necessary to activate the sonosensitiser. Cavitation can be either stable, with a consistent oscillation of bubble size under a low-pressure sound field, or inertial, where microbubbles experience violent oscillations. Sonosensitisers are non-toxic on their own; however, when ‘activated’ by cavitation and ultrasound, they release reactive oxygen species (ROS), which are toxic free radicals (Figure 2) - highly reactive atoms or molecules with at least one unpaired electron in their outer shell. These ROS can readily oxidise cellular components nearby, effectively targeting and destroying tumours.

Sonosensitisers
There are many different sonosensitisers. One of which are porphyrin-based sensitisers, which were initially used as photosensitisers (reactive to light) in photodynamic therapy (Figure 3). These molecules release single oxygen atoms or hydroxyl radicals when exposed to light and ultrasound, providing cytotoxic effects, leading to cell death. However, the result of porphyrin-based sensitisers is not as local as desired SDT treatment; The destructive and widespread damages occur in nearby non-targeted tissues as well, resulting in a less localised treatment.
On the other hand, Xanthene-based sensitisers (Figure 4) have proven to be more successful. In vitro studies have shown promising cytotoxic results using these sensitisers by producing ROS. The more localised damage makes these sensitisers more targeted to tumours, even though more research is necessary to improve its potential in vivo since Xanthene structures are quickly processed by the liver and excreted.
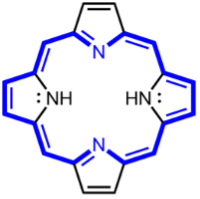
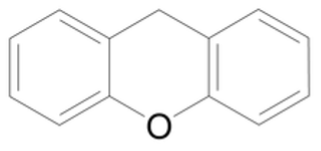
Sonoluminescence and Pyrolysis
The process of cavitation in the generation of ROS can be split into two primary mechanisms: Sonoluminescence and pyrolysis. Sonoluminescence occurs when the cavitation around the surface of cancer cells provides sufficient energy, by the collapse of cavitating microbubbles, to initiate the formation of light. Since many sonosensitisers are derived from photosensitisers, they are sensitive to both light and ultrasound. Consequently, this sonoluminescence activates the sensitiser, shifting it from its ground state to an excited state with higher energy. As the activated sonosensitiser returns to its ground state, the released energy can be transferred to surrounding oxygen molecules to produce large quantities of ROS, as mentioned earlier, including oxygen ions, hydrogen peroxide and singlet oxygen (Figure 5).
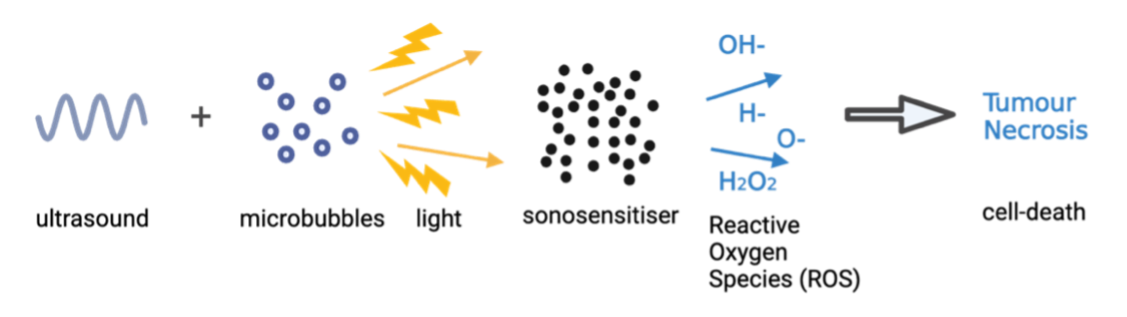
Another mechanism in action as a result of cavitation is pyrolysis - decomposition brought about by high temperatures. It is believed that the inertial cavitation - in other words the microbubbles rapidly collapsing - produces a shockwave effect, generating extreme amounts of thermal energy. The rapid temperature rise thermally decomposes the sonosensitisers in tumour cells, producing ROS vital for SDT. This pyrolysis within the cavitation microbubbles will produce H+ and OH−, ultimately leading to mitochondrial damage and tumour necrosis.
Applications of Sonodynamic Therapy
SDT has been researched most commonly to combat cancers and atherosclerosis such as breast cancer, pancreatic cancer, liver, and spinal sarcomas. Currently, no FDA approved clinical applications of SDT exist, but SDT has been used in clinical trials and applied in combination with other therapies.
Glioblastoma (GBM) is a challenging central nervous system disease to treat and has high rates of recurrence and mortality. The median GBM survival time is 12-18 months, with only 25% of patients surviving more than one year. Its characteristics of being highly invasive and undergoing heterogeneous genetic mutation, as well as the presence of the blood-brain barrier (BBB), have reduced the efficacy of current GBM treatment.
Traditional methods of treating GBM include surgery, chemotherapy and radiotherapy. However, despite these treatments being somewhat successful and efficient, they often result in systemic toxicity, low tumour selectivity and long-term side effects which begs the need for other treatments. The use of SDT is a promising method for treating GBM because it is non-invasive and more tumour specific. The BBB in GBM tumours can block chemotherapeutical drugs from reaching these cancerous cells. However, research in the past decade has shown that low-intensity ultra-sound waves, used in SDT, have the ability to temporarily open up the BBB which automatically reseals within 24 hours. This is through the cavitation effect in microbubbles which forces apart the protective endothelial cells that line in blood vessels in the brain (Figure 6). The opening of the BBB can greatly facilitate nanoparticles, such as drugs, in accumulating in tumour sites. The utilisation of ultrasound waves to open the BBB is widely explored to promote amyloid removal to treat Alzheimer’s disease.
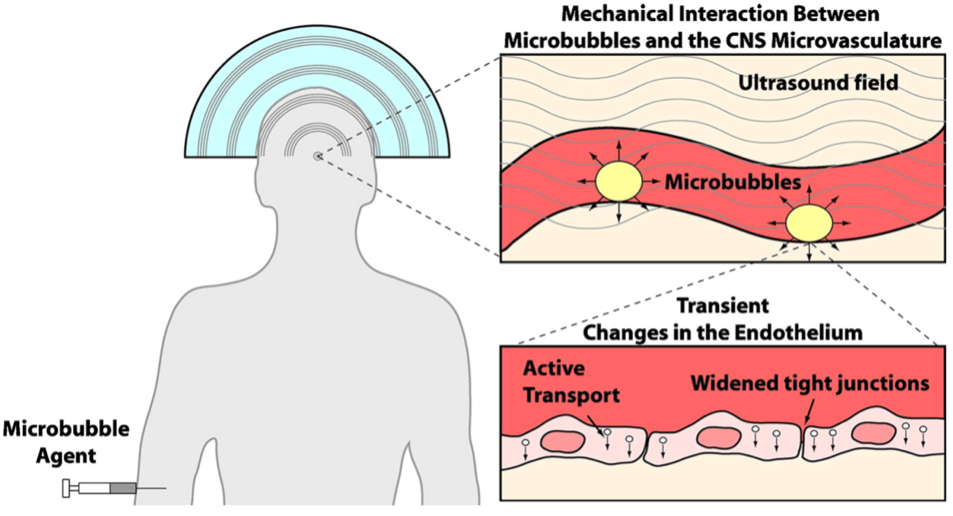
Pre-administered microbubbles facilitate the nano-sensitiser entry into tumorous cells in the brain (Figure 6). Patients receive a dose of the 5-Aminolevulinic acid (5-ALA), fluorescein, rose Bengal, titanium dioxide (TiO2) and other chemicals. Upon activation by ultrasound, ROS generation in by the sonosensitiser 5-ALA in GBM cells result in a decrease in mitochondrial membrane potential (MMP), which is a decrease in electrical charge difference across the inner membrane of mitochondria, as well as mitochondrial swelling (Figure 7). At the same time, the degradation of endoplasmic reticulum Ca2+ ATPase (SERCA2) can increase levels of cytoplasmic Ca2+ (Figure 7). This synergetic molecular mechanism leads to apoptosis (programmed cell death), through sonoluminescence and the pyrolysis effect of microbubbles.
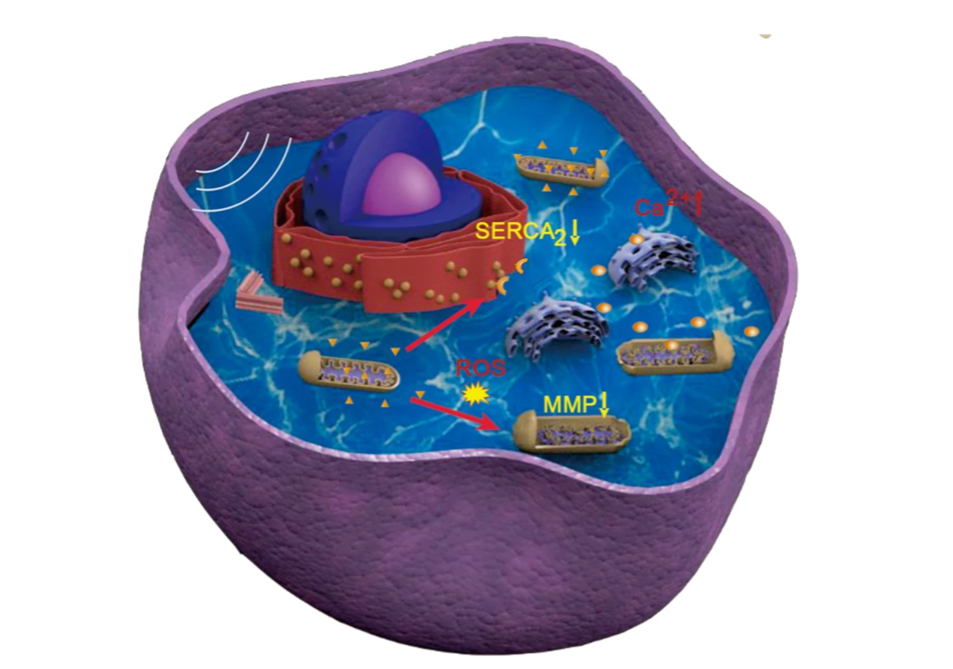
Combination With Other Therapies
SDT can be combined with other mainstream therapies such as chemotherapy and immunotherapy to create synergistic effects. Chemotherapy is a common cancer treatment where chemotherapeutic drugs, which are cytotoxic and interfere with cell mitosis, are administered intravenously or orally. However, cells which undergo mitosis, including bone marrow, digestive tract and hair follicles, are damaged, creating widespread side effects. For GBM, the presence of the BBB hinders the drug intake by tumorous cells, with only Temozolomide (TMZ) and bevacizumab being viable options. Therefore, integrating sonosensitisers with chemotherapeutic agents can help them specifically accumulate in tumour sites through the use of SDT, which opens up the BBB temporarily (Figure 6). Moreover, SDT can activate mitochondrial caspase apoptosis pathway which, in turn, increases tumour sensitivity to chemotherapeutic drugs. In other words, the synergistic interaction between sonodynamic therapy and chemotherapy improves chemosensitivity in tumours and SDT-induced apoptosis, leading to a greater efficacy of the cancer treatment.
SDT has also been combined with immunotherapy, a cancer therapy treatment which works by activating the immune system, including immune checkpoint blockade therapy, cytokine therapy, and cancer vaccines. SDT causes direct mechanical damage to cancer cells by pyrolysis, and also promotes immunogenic cell death (ICD), a form of cell death that eliminates affected cells and also elicits an immune response that helps the body recognise and attack similar tumour cells. The ROS generated during SDT triggers pathways that activate the immune system, leading to enhanced antitumour immune responses.
Conclusion
Sonodynamic therapy, a non-invasive treatment developed from photodynamic therapy, can kill tumour cells specifically and selectively. It has shown to be effective at selectively targeting tumour sites and eliminate malignant cells without widespread effect; ultrasound can generate sonoluminescence through cavitation, activating sonosensitisers to generate ROS, which induce apoptosis of cancerous cells. Additionally, shock waves and mechanical stress generated by acoustic cavitation can cause damage to mitochondria in cancerous cells. Recent studies have shown that it has the potential to boost effects of other cancer treatment strategies as low-intensity applications of ultrasound are non-toxic. However, more work and research need to be done before sonodynamic therapy is accepted as an adjuvant or replacement method for traditional cancer treatment, and a greater understanding of the mechanisms underlying the generation of reactive oxygen species induced by ultrasound waves will enable the design of more effective sonosensitisers. The strategy of combining sonodynamic therapy with chemotherapy and immunotherapy is gaining more legitimacy as more studies have validated their synergistic effects. Hence, sonodynamic therapy can have significant impact in the future of cancer therapy and research and can improve existing treatments.