The Chernobyl Disaster
Thirty-six years after the Chernobyl disaster, the chilling tale of the world's worst nuclear catastrophe continues to haunt us. Human error, Cold War secrecy, and flawed Soviet reactor design converged to unleash an unprecedented disaster. The harrowing aftermath – radioactive fallout, long-term he
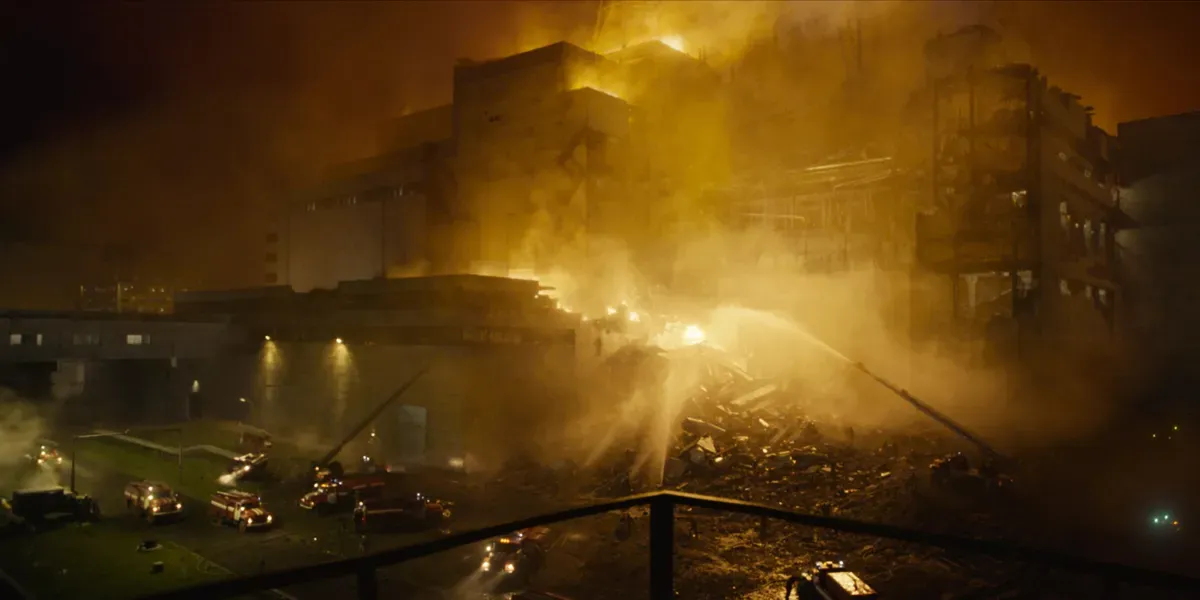
Legacy articles aren't reviewed and may be incorrectly formatted.
The Chernobyl Disaster
Simba Shi
Almost four decades ago, the failure of a reactor in a nuclear power plant in Ukraine made the headlines and shocked the entire world. The Chernobyl nuclear disaster in April 1986 is widely known as the most devastating nuclear incident that has ever occurred in history. According to epidemiological research, clean-up workers had an increased lifetime chance of developing leukaemia, cardiovascular disease, and cataracts, as well as thyroid cancer and other non-malignant disorders. The accident was caused by a combination of a flawed Soviet reactor design as well as grave errors committed by the site's operators. It was also a direct outcome of Cold War isolation and the absence of a safety culture that followed.
Reactor design
Four nuclear reactors, all made from the RBMK-1000 design, made up the Chernobyl Power Complex, which was located about 20 kilometres south of the Belarusian border and roughly 130 kilometres north of Kiev, Ukraine. The RBMK-1000 is a graphite-moderated pressure tube reactor of Soviet design and construction, which burns fuel that has been significantly enriched (2% U-235). It is a boiling light water reactor, and there is no heat exchanger in between the two loops that supply steam to the turbines. Water injected into the bottom of the fuel channels begins to boil as it moves up the pressure tubes, generating steam that powers two turbines with a combined output of 500 MWe (Megawatt electric). The steam that powers the turbines comes from the water, which also serves as a coolant. The zirconium alloy-clad uranium dioxide fuel, which is surrounded by cooling water, is housed inside the vertical pressure tubes. The fuel channel extensions are welded to both the cover plate and the bottom plate of the core.
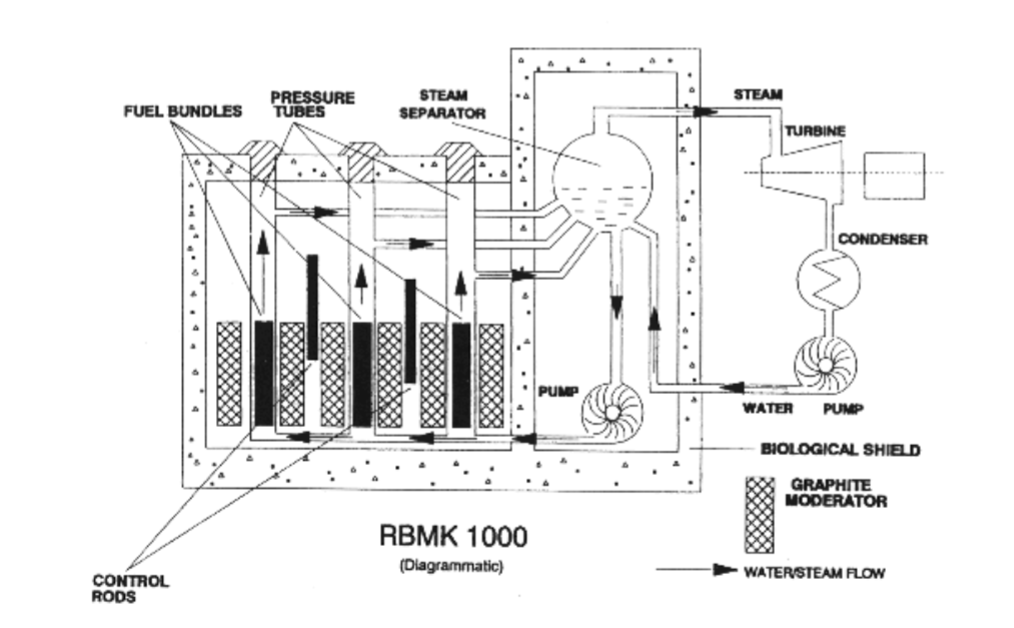
The graphite that surrounds the pressure tubes serves as the moderator; the purpose is to slow down neutrons to increase their efficiency in generating fission in the fuel. In order to keep the graphite from oxidising and to enhance the transfer of heat (generated by neutron interactions in the graphite to the fuel channel), a mixture of helium and nitrogen is circulated between the graphite blocks. About 7 metres high and 12 metres in diameter is the core itself. One of the four primary coolant circulation pumps, which are present in each of the two loops, is always on standby. By controlling 211 control rods, when lowered into the moderator, it absorbs neutrons and lowers the fission rate, therefore, regulating the reactor's reactivity or power. This reactor produces 3200 MW thermal power, or 1000 MWe. The reactor design included a number of safety devices, such as an emergency core cooling system.
The ability of the RBMK reactor to have a "positive void coefficient," in which an increase in steam bubbles (also known as "voids") is followed by an increase in core reactivity, is one of its most significant features. The neutrons that would have been absorbed by the denser water now cause more fission in the fuel as steam production in the fuel channels rises. In RBMK reactors, the vacancy coefficient predominates over other elements that make up the total power coefficient of reactivity. A new RBMK core will have a negative void coefficient since the void coefficient depends on the core's composition. The reactor's fuel burn-up, control rod arrangement, and power level at the time of the Chernobyl 4 catastrophe, however, resulted in a positive void coefficient that was sufficiently large to outweigh all other influences on the power coefficient.
Causes of accident
Prior to a scheduled shutdown on April 25, 1986, the Chernobyl 4 reactor team started preparing for a test to see how long the turbines would continue to spin and provide power to the main circulating pumps. The power from the turbine depleted too quickly during the Chernobyl test the year prior, necessitating the testing of new voltage regulator designs. The attempted test was conducted early on April 26 after a series of operator actions, including the disabling of automatic shutdown safeguards. The reactor was in a very unstable state when the operator made the decision to shut it down. The control rods' unique construction resulted in a significant power surge while they were being placed into the reactor.
Fuel fragmentation, fast steam creation, and a spike in pressure were all caused by the interaction of the extremely hot fuel with the cooling water. Due to the reactor's design features, even moderate damage to three or four fuel assemblies might and did cause the reactor to be destroyed. Due to the overpressure, the reactor's 1000-tonne cover plate partially detached, rupturing the fuel channels and blocking all of the control rods, which were only halfway down at the time. The emergency cooling circuit ruptured, causing intense steam generation to spread throughout the whole core (supplied by water pumped into the core), leading to a steam explosion and the release of fission products into the atmosphere. A second explosion occurred around two to three seconds later, spewing hot graphite and pieces from the fuel line. The graphite and fuel started a number of fires, releasing radioactivity into the surrounding environment. About 1.4 x 1019 Bq of radioactivity was emitted.
Consequences
Thirty operators and firefighters died within three months after the catastrophe destroying the Chernobyl 4 reactor, and several more people died after that. One person passed away right away, while another passed away from their injuries shortly after in the hospital. According to reports, a second person passed away at the time from a coronary thrombosis. Acute radiation syndrome (ARS) was initially identified in 237 individuals who were present at the site and involved in the clean-up; 134 further instances of ARS were later verified. 28 of these passed away within a few weeks of the accident as a result of ARS. Between 1987 and 2004, 19 additional workers passed away, however, their demises were not always related to radiation exposure. Access to the Chernobyl area has been restricted to this day due to its danger.
Although the disaster was a horrific event that affected the lives of many people, we can learn some important lessons from it and improve current disaster preparedness and management plans.
Clinical and population-based studies conducted after the Chernobyl accident have provided unique insights into the associations between radiation exposures and long-term health risks of leukaemia, CVD, and cataracts among clean-up workers. The aftermath of Chernobyl has also provided a more comprehensive understanding of thyroid diseases in those exposed to radiation as children and adolescents. One of the main things learnt from the nuclear accident is acknowledging the significance of providing timely information to the impacted populations and of having clear communication strategies built in disaster preparedness and mitigation plans.