Laser Cooling
All particles in nature are in constant motion, which makes it particularly challenging to observe phenomena on the atomic level. This problem was solved by the recent development of laser cooling techniques.
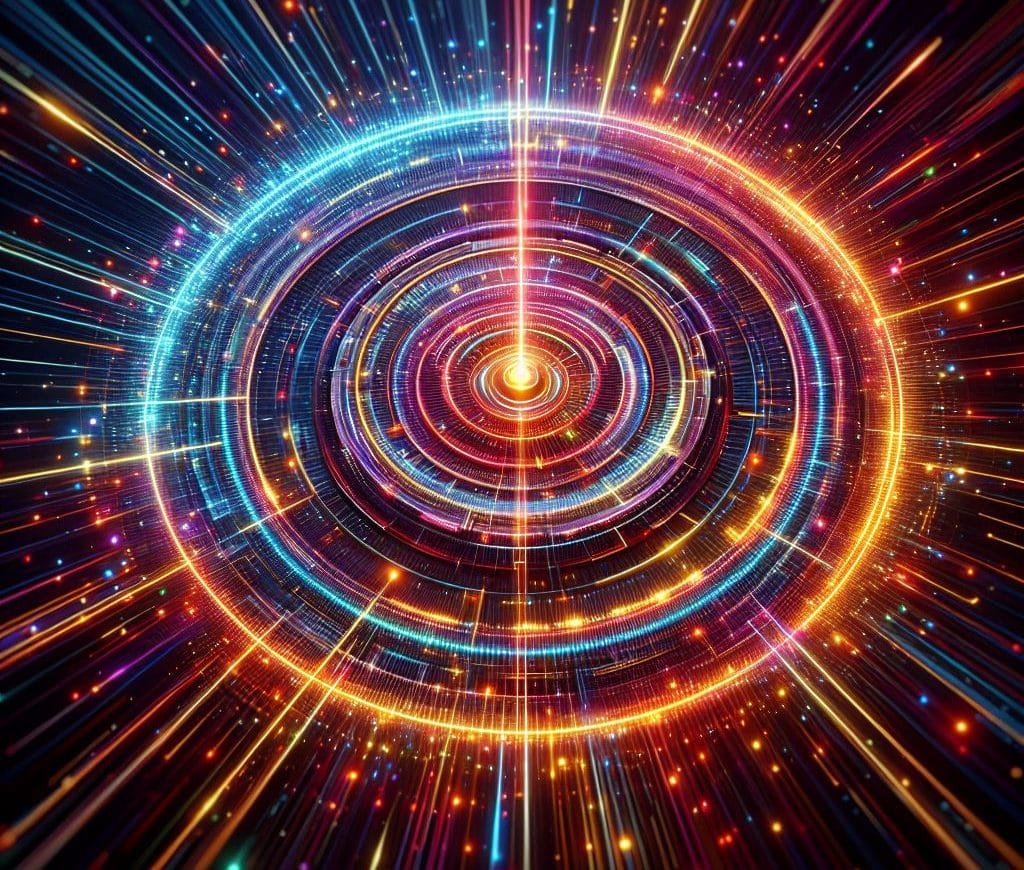
Legacy articles aren't reviewed and may be incorrectly formatted.
Laser Cooling
Warren Wong
All particles in nature are in constant motion. In solids, particles move back and forth about respective points, held together by bonds that are roughly comparable to springs. In liquids particles swoosh and slide around freely. In gases, particles move at very high speeds indeed – 1754 m/s at room temperature for hydrogen – almost 10 times the speed of a plane. This makes it particularly challenging to observe phenomena on the atomic level. This problem was solved by the recent development of laser cooling techniques.
Laser cooling works because of photons, particles that were first proposed in 1905 as finite packets of energy. Photons are massless but have a momentum given by h/λ, where h is Planck’s constant and λ is the wavelength of the wave.
Let’s say we have an atom of mass m moving to the right at a certain speed v. A laser is set up on the right, ready to fire photons towards the left, at the atom. Once the photon hits the atom the atom absorbs the photon. Because the photons are moving with a velocity that is in a direction opposite to direction of travel of the atom, deceleration of the atom occurs.
Not all photons are absorbed. As shown by the distribution below the chance that a photon is absorbed is dependent of the wavelength. The chance of absorption is greatest when laser of a certain wavelength λ is used and starts dropping when the wavelength gets higher or lower than that peak wavelength.
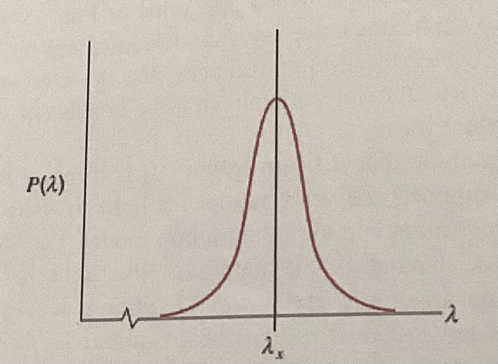
Additionally, after absorption by the atom, the release of the photon is going to change the momentum of the atom. For example, if the photon was shot backwards relative to the atom, then the atom is going to speed up due to the reaction force by the photon. However, due to the fact that the direction of photon release is random, the changes in momentum due to individual photon releases, summed together, have no net impact.
Let’s say we now have an atom moving to the left at speed v. The laser is still at the right, and fires photons to the left. The photons collide with the atom imparting it with a greater speed. This is problematic if we want to slow a bunch of atoms, all moving in random directions. Depending on the direction of travel of the atoms, they are either going to speed up or slow down.
To slow down large batches of atoms physicists needed to make atoms slow down regardless of the direction of their velocities. To do this they placed two lasers of the same wavelength that is greater than the peak wavelength, one on the left (L) and the other on the right (R), while the atoms were placed in between the two lasers.
Atoms moving to the left move into photons created by L at a higher frequency so they feel a shorter wavelength from L. Fewer photons created by R collide per second with the atoms moving to the left, so they perceive a higher wavelength from R. More photons are absorbed from L than from R as shown in the figure below, and the atom slows to a halt.
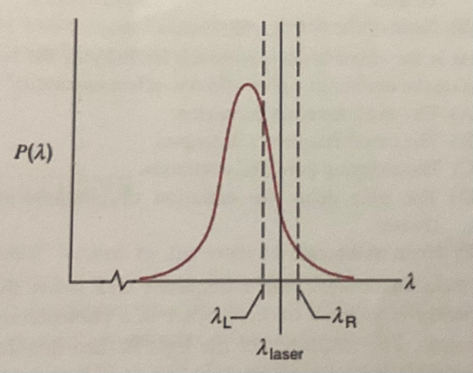
For atoms moving to the right, they would perceive a greater frequency from R, and hence a lower wavelength. They perceive a lower frequency from L and thus a higher wavelength. More photons are absorbed from R than from L. Therefore, the atom decelerates.
Note that if we used lasers of wavelengths lower than the peak, we are going to get the opposite phenomenon. Atoms are going to speed up instead of slow down.
With lasers of wavelength higher than the peak wavelength, no matter what direction the atoms move they get slowed. However, two lasers only work for atoms moving in one dimension. Molecules in a gas move into all three dimensions, so 6 lasers in opposing pairs are needed to slow gas molecules. Any molecule in the intersection of the beams can only slow down, regardless of the direction of travel. This is termed laser cooling.
The 1997 Nobel Prize in Physics went to Steven Chu, Claude Cohen-Tannoudji and William D. Philips ‘for development of methods to cool and trap atoms with laser light’. Their invention allowed for the observation of atoms now that they are at rest. Laser cooling is used, for example, in experiments involving the atomic clock; it was also used to create conditions near absolute 0, as needed for some quantum experiments. These achievements would not have been possible without laser cooling.