The Role of Nanotechnology in Targeted Drug Delivery
Take a deep dive into the growing role of Nanotechnology in Medicine, and learn about the complex technologies being developed.
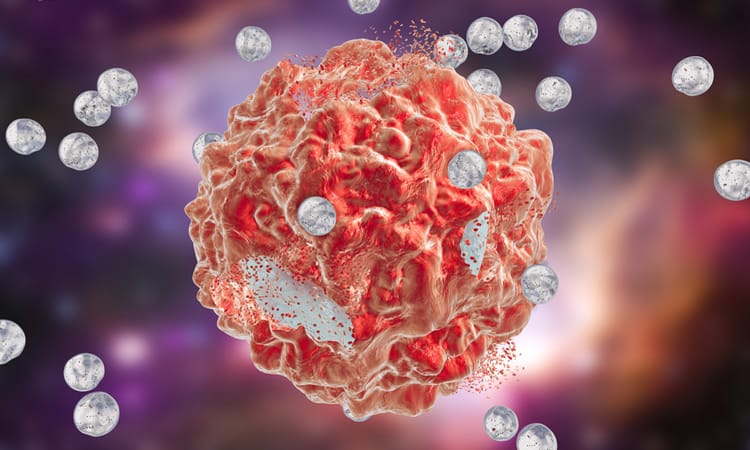
“Incurable diseases will eventually force mankind to justify disruptive nanotech and genetic engineering.” - Toba Beta
In recent years, the intersection of nanotechnology and medicine has revolutionised the way scientists approach disease treatment and prevention. As the world grappled with diseases, like Covid-19 (SARS-CoV-2), this technological frontier was central to the rapid development of effective vaccines and therapies. But what exactly is nanomedicine, and how does it function at the molecular level?
Understanding Nanomedicine
Nanotechnology, which deals with materials ranging from 1 to 100 nanometres in size, offers a promising solution to many of the limitations of conventional drug delivery - namely, biodistribution challenges and intracellular trafficking barriers. By leveraging the site-specific and target-oriented capabilities of nanomedicine, researchers are able to enhance drug efficacy while minimising systemic toxicity.
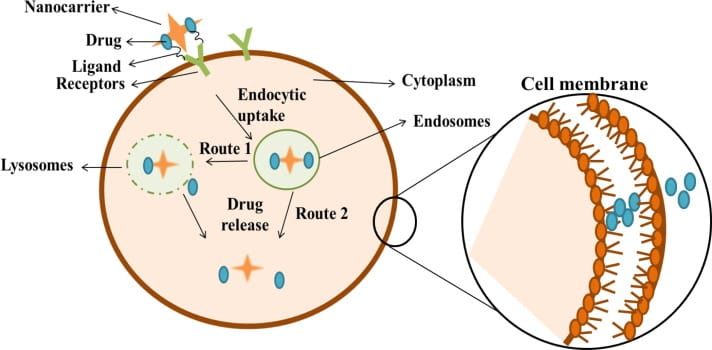
At its basis, drug delivery refers to the various approaches through which a pharmacologically active agent is transported to its target cell to treat diseases or health conditions. The mechanism for targeted drug delivery follows a three-step process (Fig. 1). First, a nanocarrier binds to target cell receptors through multivalent receptor-ligand interactions[^ Multivalent receptor-ligand interactions occur when multiple ligands bind to multiple sites on a receptor, creating a cooperative effect that greatly increases the bonding affinity and specificity of the interaction.]. Then, the nanocarrier enters the cell through endocytosis - the cellular process of internalising substances. Finally, the drug is released within the cell, often diffusing out of the nanocarrier through a porous matrix or shell. Alternative release mechanisms can occur too: nano carriers may degrade due to biological conditions, enzymes may break specific bonds within the carrier, or environmental factors like pH and temperature change may trigger drug release.
COVID-19 and the Role of Lipid Nanoparticles
When the World Health Organisation (WHO) declared the COVID-19 outbreak a pandemic, researchers around the globe launched massive efforts to combat this infectious disease. The technology of lipid nanoparticles was crucial in overcoming challenges related to vaccine efficacy and delivery as without this advancement, the mRNA vaccines of today, such as those from Moderna and Pfizer-BioNTech, would have struggled with stability, bioavailability and immune response activation. It both promoted sustained drug release and the immunogenicity[^ The ability of a substance that contains antigens to cause the body to make an immune response against that substance.] of the antigen.
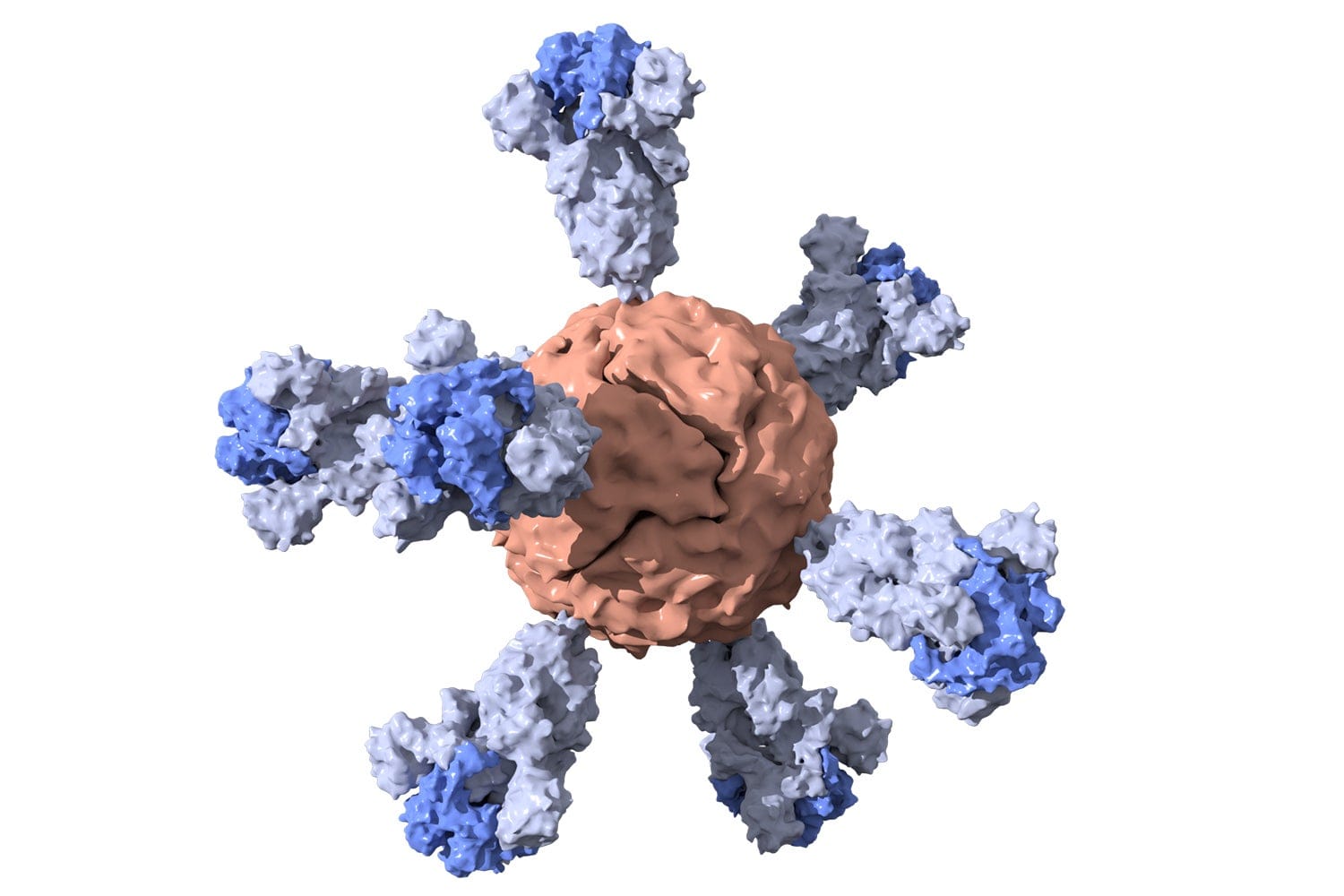
Lipid nanoparticles, the most common class of FDA-approved nanomedicine, are spherical platforms comprised of at least one lipid bilayer surrounding an internal aqueous compartment. These structures offer distinct advantages, including biocompatibility (the ability to interact with biological systems without provoking adverse immune responses), high bioavailability (the proportion of the administered drug that reaches systemic circulation[^ Systemic circulation refers to the network of blood vessels that delivers oxygen, nutrients and therapeutic agents throughout the body, ensuring that the administered drug reaches target tissues and organs in its active form.] in an active form), and a capacity to carry large therapeutic payloads.
Although SARS-CoV-2 was not the first pathogen to be treated with a nanomaterial-based carrier (Fig. 2), it certainly highlighted the potential of nanomedicine for antiviral drug synthesis. Since the pandemic, applications of nanoparticles in chemotherapy and immunotherapy has grown, and their use in clinical diagnostics has been further explored. It is becoming increasingly clear that future of drug carriers lies at the nanoscopic level - but how do different nanoparticle systems function, and what challenges remain?
Advances in Nanoparticle Design
The success of a nanoparticle delivery system depends on its physicochemical properties, which dictate its interactions with biological barriers. While nanoparticles have evolved over the years, one major challenge has persisted: heterogeneity. Early nanoparticle research relied on animal models, but physiological differences between animal and human species resulted in a translational gap. While nanomedicines demonstrated efficacy in animal studies, they did not always translate to human success due to unforeseen biological variations.
Even today, under-explored heterogeneity is a huge problem. Any slight difference in the growth or structure of diseased tissue can alter distribution and functionality. The Precision Medicine Initiative (PMI), launched in 2015, seeks to address this by classifying patients based on genetic profiles, comorbidities and environmental exposures. This stratification enables the development of nanoparticles tailored to specific patient subsets, thereby optimising them for more personalised types of drug delivery and improving the efficacy of precision medicine therapies.
Classes of Nanoparticles in Medicine
As was mentioned, lipid particles have been instrumental in vaccine development. However, other classes of nanoparticles (Fig. 3) also offer diverse applications in drug delivery and diagnostics:
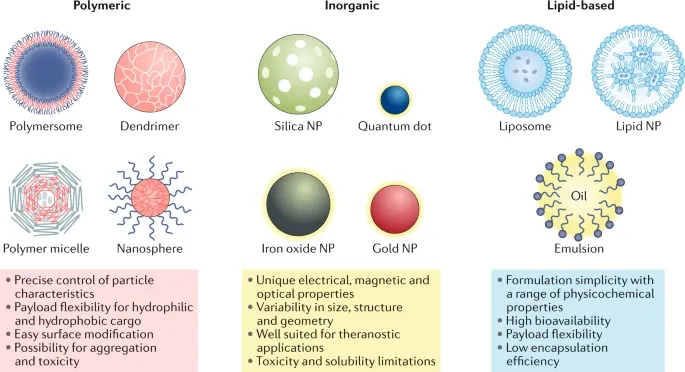
- Polymeric Nanoparticles: Synthesised from natural or synthetic materials (as well as monomers or performed polymers), these can take the form of nanocapsules (hollow spheres) or nanospheres (solid matrices). Their versatility allows for the encapsulation of both hydrophilic and hydrophobic compounds and cargos of differing molecular weights, broadening the range of payloads they can deliver.
- Inorganic Nanoparticles: Used primarily in imaging and diagnostics, these nanoparticles leverage unique magnetic, radioactive and plasmonic[^ Plasmonic relates the unique optical properties exhibited by nanoparticles, especially metallic ones, that enable them to strongly interact with light resulting in enhanced scattering or absorption, which can be used to increase contrast and improve the detection of specific biological targets.] properties. For example, iron oxide nanoparticles improve PET[^ Positron Emission Tomography (PET). Allows for the visualisation of metabolic activity in tissues, which is crucial for diagnosing diseases like cancer.] and SPECT[^ Single Photon Emission Computed Tomography (SPECT). Uses gamma-emitting radiotracers to capture detailed 3D images of physiological processes, providing insight into blood flow, tissue function and the localisation of disease.] imaging by enabling real-time visualisation of metabolic processes. Given these nanoparticles can accumulate in specific areas of interest, improving contrast and clarifying the locations of diseased and healthy tissues, they have also found a place in MRI scans.
- Quantum Dots: These semiconductor nanocrystals are a specific example of the inorganic class and have gained prominence in bioimaging as a result of their high luminescence. The 2023 Nobel Prize in Chemistry was awarded to Moungi Bawendi and Aleksey Yekimov for their pioneering work on quantum dots, revealing their potential.
Challenges and Ethical Considerations
While nanotechnology has proved significantly advantageous to pharmaceutical development, there are not without risks. Some, such as quantum dots, exhibit cytotoxic effects[^ Cytotoxic effects refer to the harmful impact of substances or agents on cells, typically leading to cell damage or death by disrupting cellular structures, functions or metabolism.] or provoke immune responses, leading to potential toxicity in healthy tissue. Achieving precise in vivo targeting remains challenging, as nanoparticles must navigate complex biological environments without accumulating in unintended regions.
Economic feasibility is another barrier to widespread nanomedicine adoption. The high costs associated with research, development and manufacturing make traditional drug formulations - such as tablets, capsules and injectable solutions - the most cost-effective option for many pharmaceutical companies.
The Future of Nanomedicine
Despite these challenges, nanomedicine's potential is vast. The ability to design nanoparticles for encapsulating therapeutic agents and directing them to tumour sites could revolutionise cancer treatment, addressing issues of poor solubility and non-specific bio-distribution. Ongoing research aims to refine our understanding of how nanoparticles interact with immune systems, paving the way for safer and more effective therapies.
“If we can reduce the cost and improve the quality of medical technology through advances in nanotechnology, we can more widely address the medical conditions that are prevalent and reduce the level of human suffering.” — Ralph Merkle
If advancements continue at their current rate, nanomedicine could become a standard practice in healthcare, offering personalised, targeted and efficient treatment options. Hopefully, we will eventually be able to understand and fully exploit their therapeutical capabilities to ensure precision medicine becomes more accessible.
Bibliography
Alghamdi, Maha Ali, et al. "The Promise of Nanotechnology in Personalized Medicine." Journal of Personalized Medicine, vol. 12, no. 5, 2022, p. 673, https://doi.org/10.3390/jpm12050673.
Sultana, Afreen, et al. "Nano-Based Drug Delivery Systems: Conventional Drug Delivery Routes, Recent Developments and Future Prospects." Medical Innovations & Developments, vol. 2, 2022, p. 100134, https://doi.org/10.1016/j.medidd.2022.100134.
Loh, X. J., Abdul Karim, A., and Owh, C. “Nanotechnology and the Future of Diagnostics and Treatment.” Frontiers in Nanotechnology, vol. 2, 2020, article 589541, https://doi.org/10.3389/fnano.2020.589541.
Madar, M. "The Effectiveness of Nanoparticles in Chemotherapy." Drug Target Review, 19 Dec. 2023, www.drugtargetreview.com/article/112774/the-effectiveness-of-nanoparticles-in-chemotherapy/.
Mitchell, Michael J., et al. "Engineering Precision Nanoparticles for Drug Delivery." Nature Reviews Drug Discovery, vol. 20, 2021, pp. 101–124. Nature Publishing Group, https://www.nature.com/articles/s41573-020-0090-8.